The debate concerning the origin of our minds stems back to the diverging opinions of Darwin (1871) and Wallace (1870). When Charles Darwin first discussed the evolution of our seemingly unique cognitive faculties, he proposed that there is “no fundamental difference between man and the higher mammals in their mental faculties.” (Darwin, 1871, pg. 66). Conversely, Wallace was suspicious of whether natural selection alone could have shaped the human mind, writing: “[…] that the same law which appears to have sufficed for the development of animals, has been alone the cause of man’s superior mental nature, […] will, I have no doubt, be overruled and explained away. But I venture to think they will nevertheless maintain their ground, and that they can only be met by the discovery of new facts or new laws, of a nature very different from any yet known to us.” In the intervening years, the debate surrounding the degree of continuity between animal and human minds still rages on in contemporary discussions (Bolhuis & Wynne, 2009; Penn, Holyoak & Povinelli, 2009).
The notion that we can identify a sequence of adaptations accounting for the evolution of minds in animals stems from research into social cognition, particularly surrounding Premack & Woodruff’s (1978) concept of a Theory of Mind. Not only has this research focused on species closely related to humans, such as chimpanzees, but it also touches upon cognition in birds, dogs, fish and many other animals (Bolhuis & Wynne, 2009). This approach frequently elicits interesting findings, and in some instances suggests applying a strictly evolutionary approach to cognition may not yield the most relevant results (Topál, in press).
Another major stepping stone is found in approaches to the emergence of human cognition. Pioneering insights by the likes of Leda Cosmides and John Tooby (1997) have certainly shaped the direction of research, with them suggesting our mind is massively modular and evolved as a direct result of selective pressures during the Stone Age. As we shall see, this approach is not only very difficult to investigate, but potentially it ignores other explanations for shaping our cognition that argues our mind may be better adapted to contemporary society than previously thought (Bolhuis & Wynne, 2009).
Lodged in-between these explanations are possible solutions and potential directions for future research, particularly in the realms of comparative social cognition (Emery & Clayton, 2009) and models linking genes, neurobiology and social behaviour (Robinson, Fernald & Clayton, 2008).
Adaptive Minds: Genes, Neurobiology, and Social Behaviour
Even though there are differing degrees of behavioural complexity across various animal domains, can a sequence of adaptations be established? Although challenging, Robinson, Fernald & Clayton (2008) provide a useful organisational heuristic outlining two vectors of influence for understanding social behaviour in the context of genes and neurobiology: “Vector 1 describes how social information leads to changes in brain gene expression, brain function, and social behaviour, and Vector 2 describes how genetic variation between individuals leads to variation in social behaviour.” (pg. 896).
Highly relevant to social behaviour influencing brain gene expression is a gene known as egr1 (ibid). Found to play a role in many social species, including zebra finch (Mello, 2005), cichlid fish (Burmeister, Jarvis & Fernald, 2005) and prarie voles (Donaldson & Young, 2008), egr1 is a transcription factor-encoding gene that remains responsive throughout the lifetime of an organism (Robinson, Fernald & Clayton, 2008). In male zebra finch (Taeniopygia guttata), for example, “[…] the singing of another male bird induces egr1 expression in a specific subregion of the auditory forebrain devoted to hearing.” (ibid, pg. 896).
Offering an even more relevant example for the role of genes in social animals are cichlid fish (Astatotilapia burtoni), where dominance hierarchies govern most social interactions (ibid). Here, rank is determined through both male competitive fighting and observations of these fights, suggesting these fish are capable of transitive inference (Grosenick, Clement & Fernald, 2007). When an alpha male is no longer part of the group of which he is dominant, a subordinate male will begin to display dominant-like behaviour. Interesting, the onset of this behaviour results in changes in body colouration, triggering egr1, “[…] specifically in the hypothalamic anterior preoptic area in neurons containing gonadotropin-releasing hormone (GnRH), a peptide critical for reproduction.” (Robinson, Fernald & Clayton, 2008, pg. 897).
The relevance of GnRH in cichlid fish is that dominants are biologically more fertile than subordinates, and therefore enjoy a greater reproductive success (ibid). Furthermore, egr1 is just one of many genes known to be expressed due to changes in behaviour, which in itself is caused through the wider context of social information (ibid). As Robinson et al. (2008) state, “[…] it is now clear that responses to social stimuli can be massive, involving hundreds or thousands of genes and perhaps many different brain regions at once.” (pg. 897).
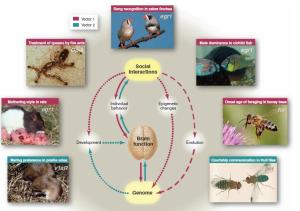
Other social influences include heritable epigenetic effects, adding to the complexity of potential influences on brain gene expression (ibid). Maternal behaviour in rats is one such instance, where licking and grooming “[…] regulate the development of endocrine, emotional and cognitive responses to stress.” (Champagne et al., 2003, pg. 329). In addition, these effects of social information must also account for individual genetic variation, which can also lead to differences in the social behaviour and cognitive functioning within a group or species (Robinson, Fernald & Clayton, 2008).
As already mentioned, the second vector accounts for what is essentially the reverse situation, where genes influence the behaviour of a particular species. A prominent case is that of foxp2, a regulatory gene that is implicated in numerous social roles across vertebrates (ibid), including human speech (Enard et al., 2002), song learning/production in zebra finch (Haesler et al., 2007), echolocation in bats (Li et al., 2007), and more broadly, sensory-motor coordination (ibid). According to Robinson et al. (2008), accounting for the link between genes like foxp2 and social behaviours is understood through an evolutionary approach: “Through selection, genes may evolve according to their effects on a social behavior, even if their mechanistic roles in the neural expression of that behavior are subtle and indirect.” (pg. 899).
Humans: Modern skulls, stone age minds?
Although significant advances in understanding animal cognition have been made in the last few decades, there is still considerable debate over the capabilities animals possess. Looking at animal competencies appears to reveal they are mostly restricted to a single goal, in contrast to the numerous goals subserved by human cognition (Premack, 2007). Even if there is not an apparent continuity between human and animal minds, both behaviourally and biologically (ibid), it is highly relevant to consider the selective processes underlying the development of our human minds.
Under the assumption of some researchers (Cosmides & Tooby, 1997; Pinker, 2002), our minds are very much like organs, in that they consist of a collection of functionally specialised circuitry. Like our hearts and livers, which evolved to pump blood and detoxify blood respectively (Cosmides & Tooby, 1997), our brain contains neural circuitry specialised for a whole host of specific processes, including but not limited to vision, hearing, and sexual attraction (ibid). One set of dedicated circuitry is often known as a module (ibid).
Indeed, there is little argument over the minds of humans and animals being separated into distinct processing pathways and regions for aspects like vision and hearing (Skoyles, 2009). However, the debate gets more controversial when cognitive functions formerly believed to be underpinned by general purpose processing, such as learning, reasoning, and decision making, are argued to be of a modular basis (Cosmides & Tooby, 1997). As these arguments are made under an evolutionary adaptive framework, our modules are “[…] calibrated to the environments in which they evolved, and they embody information about the stably recurring properties of these ancestral worlds.” (ibid, principle 4).
If natural selection is the driving process behind shaping our brain, then the circuitry which evolved on the human lineage is not adapted to our modern environment, rather it is evolved for a small and nomadic hunter-gather society (ibid). This reasoning is on the basis of natural selection being conventionally thought of as a slow process, taking tens of thousands of years to make even the smallest of changes (ibid). Our species has only developed agriculture in the last 10,000 years, with most of our hunter-gather pre-history consuming approximately 99% of humanity’s time on the planet (ibid). In the words of Stephen Jay Gould, “There’s been no biological change in humans in 40,000 or 50,000 years. Everything we call culture and civilization we’ve built with the same body and brain.” (Cochran & Harpending, 2009, pg. 1).
The proposition of humans having modern skulls that house a Stone Age mind (Cosmides & Tooby, 1997) has been thoroughly explored in the past two decades, with adaptive modules being attributed to numerous forms of processing (Adolphs, 2009). Face processing (Kanwisher & Yovel, 2006), kinship perception (Lieberman et al., 2007), and cheat-detection (Trivers, 1971; Cosmides & Tooby, 1992) are all cases where these modules are evolutionary adapted to process social information (Adolphs, 2009). Another recent proposal suggests that common sets of specialisations, sometimes known as supermodules, exist to deal with broader sets of related behaviours (Penn et al., 2008), such as language (Clark, 2006) and Theory of Mind (Brüne & Brüne-Cohrs, 2005).
Despite the strong case for modular processing, the contentious issue surrounding whether or not cognition is shaped by natural selection (Bolhuis & Wynne, 2009) allows for a relatively specific question to be asked: are modules evolutionary adaptive responses to ancestral environments?
First, the commonly held assumption of adaptive responses taking a long time to emerge is not necessarily correct for the human lineage. In a paper by Hawks et al. (2007) they demonstrate how vastly increasing populations over the past 50,000 years have led to significantly more new adaptive mutations. Looking at the HapMap datasets, the group argue recent selection has occurred at an increasing rate during recent history, stating: “To the extent that new adaptive alleles continued to reflect demographic growth, the Neolithic and later periods would have experienced a rate of adaptive evolution more than 100 times higher than characterized [by] most of human evolution.” (ibid, pg. 3).
Although demography is paramount in driving adaptive evolution, human populations were interacting with cultural and ecological changes (ibid). Some recent evolutionary changes include: skeleton morphology (Larsen, 1995), skin pigmentation (Ding et al., 2002), and disease resistance (Wang et al., 2006) among many others. Also, counter to Gould’s assertion of no biological change taking place in the last 50,000 years (see above), the authors claim some of the most radical adaptations emerged subsequent to the inception of agriculture (Hawks et al., 2007). For instance, selection on the lactase gene is the result of selective pressures from recent subsistence and dietary changes (Bersaglieri et al., 2004).
Of course, all of these examples of recent adaptive evolution are unrelated to cognition. There are examples of cognition-related genes believed to be undergoing natural selection (Sabeti et al., 2006; but see Fuli Yu et al., 2007), such as the derived haplotypes of ASPM and microcephalin (Dediu, 2008). More recently, a mutated version of a gene (DAB1) involved in organising brain cells, particularly those found in areas that utilise higher cognitive functions, appears to have undergone selection in Chinese populations (Williamson, 2007). Still, we know little about the role of these intensely studied genes, and by extension their adaptive advantage remains elusive.
Even if populations are still adapting, this may bolster the case for adaptive modules to modern social pressures. One possible case is the Visual Word Form Area (VWFA), a brain specialisation that is “[…] essential to rapid reading ability because it enhances perception of words by becoming specifically tuned to recurring properties of a writing system.” (McCandliss et al., 2003, pg. 293). Like arguments for adaptive modular face processing in the Fusiform Face Area (see Adolphs, 2009), the same logic can be extended and applied to the VWFA. As McCandliss and colleagues (2003) note, this finding “[…] poses a challenge for evolutionary accounts involving innate mechanisms for functional brain organization.” (pg. 293).
Accounting for the existence of the VWFA, and cognitive modules in general, may instead be more rooted in developmental than evolutionary explanations (Skoyles, 2009; Bolhuis & Wynne, 2009). Bolhuis & Wynne (2009), for example, argue the gene-constructivist approach adopted by the likes of Cosmides & Tooby (1997) overlooks cultural processes in shaping complex cognition. Supporting this notion are discoveries concerning the role of neuro plasticity (cf. Skoyles, 2009), which “[…] refers to the capacity of neural circuits to shift from one information processing operation to another to which it may, or may not, be related but to which it is distinct” (ibid, pg. 8).
Still, an amnesty between Darwin’s and Wallace’s views may be reachable: humans minds cannot be accounted for solely by biological evolution, but rather they are the products of biological and cultural evolution. However, expanding on this notion must wait until the next post in this series.
Major References
Robinson, G., Fernald, R., & Clayton, D. (2008). Genes and Social Behavior Science, 322 (5903), 896-900 DOI: 10.1126/science.1159277
Hawks, J., Wang, E., Cochran, G., Harpending, H., & Moyzis, R. (2007). Recent acceleration of human adaptive evolution Proceedings of the National Academy of Sciences, 104 (52), 20753-20758 DOI: 10.1073/pnas.0707650104
Bolhuis, J., & Wynne, C. (2009). Can evolution explain how minds work? Nature, 458 (7240), 832-833 DOI: 10.1038/458832a
John Skoyles (2009). The paleoanthropological implications of neural plasticity CogPrints: http://cogprints.org/6357/
This is a truly impressive analysis, very detailed and pulling a wide range of relevant data and applying it usefully to core questions. Thank you very much for this great example of clear and powerful science writing.